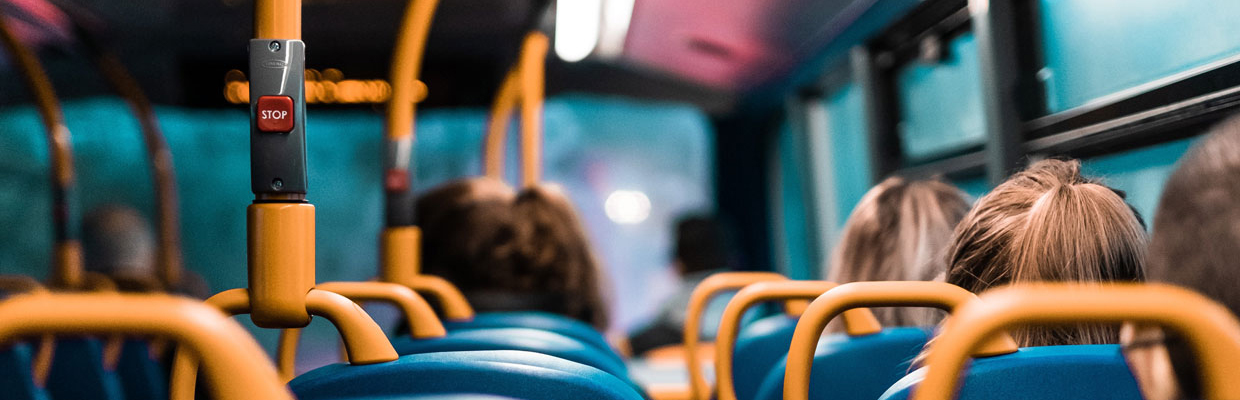
Economic rebound effects
Reading time: 27 minutes
Paul Brockway and Matthew Dickinson
Our study has shown that there is a substantial potential for energy demand reduction in the UK (over 50% of final energy demand by 2050). Without large energy demand reductions it is impossible for the UK to meet its net-zero carbon budget by 2050.
Rebound effects are not modelled explicitly within the study’s scenarios, however, reducing energy demand creates the possibility for rebound effects that could increase energy demand and reduce the effectiveness of any policies. This explainer is intended to discuss the latest research on rebound effects and highlight the potential effects introduced by assumptions within the study’s scenarios, including the potential scale of any rebound effects, and what assumptions or future actions can be undertaken to reduce those effects.
This study is intended to model the best-case reduction in energy demand, whilst either maintaining or improving standards of living. Therefore, although rebound effects may reduce the realised reduction in energy demand, this should not detract from the overarching conclusion of the study, that reducing energy demand is fundamental to meeting the UK’s net-zero targets.
This explainer has considered all assumptions within both the Shift demand and the Transform demand scenarios. There is a wealth of research on rebound effects, however, these are all context specific, therefore in most cases they cannot be directly related to the assumption within this study. However, this explainer is intended to estimate the potential ranges of rebound magnitudes for different sectors, rather than find an exact value and identify what gaps remain in estimating rebounds.
The rest of this explainer is structured as follows. First, we provide an overview of what rebound effects are before we set out rebound magnitudes estimated for different sectors (including mobility, nutrition, buildings, materials and products). We then discuss other aspects such as digitalisation, and finally we provide our conclusions.
Rebound effects
In the context of energy demand reduction, ’rebound effects’ refers to the behavioural responses to changes that are intended to reduce energy consumption that subsequently increase energy demand and reduce the potential energy savings (Druckman et al., 2011). There are various types of rebound effects, including ‘direct rebounds’, ‘indirect rebounds’ and ‘macroeconomic rebounds’.
For example, more fuel-efficient cars will reduce the demand for energy in order to drive a certain number of miles, however, if this encourages people to drive further distances (for example, due to lower fuel costs) this will increase energy demand and offset some of the efficiency savings – this is considered a ‘direct rebound’ (Sorrell, 2007). ‘Indirect rebounds’ occur when a certain change increases the demand for other energy services (Raynaud et al., 2016). For example, money saved from more fuel-efficient cars may be spent on other energy services (Sorrell 2007). Combined, direct and indirect rebound effects are ‘micro-economic rebound effects’ (Vivanco et al., 2018). Finally, ‘macroeconomic rebounds’ are the changes that occur across the entire economy, for example widespread increases in energy efficiency may encourage economic growth which will increase the demand for energy (Vivanco et al., 2018).
Although a large amount of the research on rebound effects is concerned with the rebound from efficiency gains (Sorrell, 2007), other changes can also produce rebounds, for example a reduction in household food costs due to less food waste encouraging spending on other energy services (Druckman et al., 2011).
Rebound effects are usually reported as a percentage of the expected energy savings that are offset (Sorrell, 2007). Many of the studies used here report the rebound effect in either the changes in energy demand/usage or changes in greenhouse gas (GHG) emissions. However, GHG emissions are determined by other factors that change over time such as the carbon intensity of energy generation. Results reported in terms of GHG emissions therefore cannot be directly compared to the results of the energy demand scenarios. The results of rebound studies also depend on the different methodologies used between studies for example, life cycle analysis (LCA) versus input-output (IO) studies. This again reduces how much results can be directly compared. However, this explainer is intended to indicate the potential size and direction of the impact on rebound effects, not to find an exact number, therefore various studies have been included to help this discussion.
Mobility
The main focus of the mobility sector is to reduce the need for travel through a range of measures including, better land-use planning, public transport facilities and more active travel, as well as increased rollout of electric vehicles for personal car use and road freight. Table 1 summarises some of the recent research on rebound effects in the mobility sector, which range from 10-83%.
Reference | Change | Type of rebound | Measure (e.g. GHG, energy etc.) | Rebound effect |
---|---|---|---|---|
Stapleton et al., 2017 | Personal car use | Direct | Distance travelled | 26% |
Coulombel et al., 2019 | Personal car use | Direct | CO2 emissions | 68–77% |
Gillingham, 2020 | Personal car use | Direct | Distance travelled | ~10% |
Chitnis et al., 2014 | Personal car use | Direct and indirect | CO2 emissions | 25–65% |
Bjelle et al., 2018 | Personal car use | Direct and indirect | CO2 emissions | 57–83% |
Murray, 2013 | Personal car use | Direct and indirect | CO2 emissions | 12–24% |
Druckman et al., 2011 | Personal car use | Indirect | CO2 emissions | 25% |
Vivanco et al., 2021 | Personal car use | Economy wide | CO2 emissions | ~20–50% |
Matos and Silva, 2011 | Road freight | Direct | Energy use | 24% |
De Borger and Mulalic, 2012 | Road freight | Direct and indirect | Fuel use | Short term – 10% Long term – 17% |
Sorrell and Stapleton, 2018 | Road freight | Direct | Tonnes of freight moved | 50–60% |
Certain assumptions within the mobility sector modelling will reduce the number of cars on the road – for example more car sharing journeys, more “car usership”, less commuters because of homeworking and four-day work week. This could make road transport cheaper and make car transport become more attractive (for example, less congestion, cheaper and quicker journeys etc.). There is potential for this to cause rebounds as people travel longer distances and drive more (distance effect), relocate further from urban centre and commute longer distances (relocation effect) or switch from public transport and active travel to cars (modal shift) (Coulombel et al., 2019).
There is a large variety in the rebound effects on transports, however, most studies reported rebounds of at 20-25% and some were much higher in the 60-80% range, indicating that road transport policies on their own have a large potential for moderate to significant rebounds. However, individual results are dependent on context specific factors. For example, Coulombel et al. (2019) studied the rebound effect of car sharing and found a large rebound. This study was based on Paris transport, in a city which has a well-used public transport network. Therefore, there are more people using public transport that could switch to cars if they become more attractive and increase the potential rebound from the modal effect. However, if this study was based on a rural area with high numbers of car drivers and poor public transport this rebound may be much smaller because there are less people able to switch away from public transport.
The mobility scenario modelled in the role of energy demand reduction in achieving net-zero in the UK report includes a number of policies that could reduce the rebound effect, for example improving public transport (for instance, doubling investment and integrated ticketing systems) or improving active transport options (for example, cycle lane investment) which can reduce rebound by reducing modal effect (less people swap to use cars). However, this can also create its own rebound by increasing the distance people may travel, which in the case of public transport, will generate energy demand and emissions.
The introduction of a four-day working week and more remote working through ICT could reduce the rebound effect by reducing the need for commuting, however, multiple studies have shown that on their own these policies can have significant rebound effects, sometimes over 100% (de Abreu e Silva and Melo, 2017; Budnitz et al., 2020). This is because remote workers often have much longer commutes and the lower demand for commuting travel could be offset by increases in leisure travel (Holden and Linnerud, 2011).
Several policies/assumptions in the mobility sector could make car travel less attractive – for example, by reducing road capacity (through a stop on road building and some roads being repurposed for cycle lanes) or implementing an eco-levy to increase the cost of travel. This may reduce the rebound effect significantly. Coulombel et al., 2019 found reducing road capacity reduced the rebound effect of car sharing by half, but it could also increase other costs on society – for example longer journeys, more time wasted in traffic, more noise pollution etc.
In terms of road freight, there are potential rebounds by increasing the efficiency of road freight leading to increased goods delivered and encouraging long-term economic growth. The reported rebound effects in the literature also varies across the studies quite significantly.
Overall, there is potential for large rebound effects in the mobility sector. However, a key reason that mobility rebounds are high is because policies often focus on individual attempts to reduce everyday car transport not the overall aim to reduce CO2 emissions from all travel (Holden and Linnerud, 2011). Although some individual rebounds may be high, the combination of wide ranging policies and assumptions could mitigate this rebound. There is limited data on the interaction of different policies across different regions, therefore it is difficult to predict exactly how large the rebound effect may be from the assumptions and policies modelled in the low energy scenarios within the report, without more specific research.
Nutrition
Reference | Modelled change | Type of rebound | Measure (e.g. GHG, energy etc.) | Rebound effect |
---|---|---|---|---|
Tukker et al., 2011 | Diet change | Direct and indirect | CO2 emissions | ~0% |
Wood et al., 2018 | Diet change | Direct and indirect | CO2 emissions | 25% |
Alfredsson, 2004 | Diet change | Direct and indirect | CO2 emissions and energy use | CO2 emissions – 200% Energy use – 300% |
Lenzen and Dey, 2002 | Diet change | Direct and indirect | CO2 emissions and energy use | CO2 emissions – 45–55% Energy use – 112–124% |
Grabs, 2015 | Diet change | Indirect | CO2 emissions and energy use | CO2 emissions – 49–56% Energy use – 95–104% |
Bjelle et al., 2018 | Diet change and food waste reduction | Direct and indirect | CO2 emissions | 11–16% |
Chitnis et al., 2014 | Food waste | Direct and indirect | CO2 emissions | 66–106% |
Salemdeeb et al., 2017 | Food waste | Direct and indirect | CO2 emissions | 23–59% |
Druckman et al., 2011 | Food waste | Indirect | CO2 emissions | 51% |
The nutrition sector focuses on three main changes to the quantity and type of food that is consumed in the UK, these are: reducing the average calorie intake, reducing food waste, and changes to more sustainable food choices i.e. lower meat consumption and more plant-based diets.
The main effects from these changes are likely to be indirect rebounds from re-spending money saved from diet and food waste changes on other goods and services (Grabs, 2015) as opposed to causing a direct rebound. However, this depends firstly on whether these changes save money for consumers and secondly what that re-spending goes towards.
In terms of switching to more “sustainable” diets, there is uncertainty in the nature of the rebound. Whilst some studies have shown large rebound effects (sometimes over 100%) from a change in diet (Lenzen and Dey, 2002; Alfredsson, 2004; Grabs, 2015) other studies have shown that “healthier” diets can increase the cost of food – for example Tong et al. (2018) and Tukker et al. (2011) find no direct rebounds from switching to a “healthier” diet. Additionally, the overall rebound effect is very context specific, and there are large differences in the rebound effect, depending on household wealth. In general, richer households use the money saved to spend more on leisure items with lower emissions/energy usage and therefore had smaller rebounds (Chitnis et al., 2014).
Reducing food waste has been shown to have significant rebound effects and whilst there is still substantial uncertainty, multiple studies have shown rebounds effects at over 50%. (Druckman et al., 2011; Chitnis et al., 2014; Salemdeeb et al., 2017). However, none of the studies reviewed explicitly considered macroeconomic rebounds. Transformative changes in food consumption is likely to lead to equally large changes in production, for example lower domestic demand for meat and fish could increase the amount that is exported, generating energy demand and emissions (Tukker et al., 2011).
Reducing calorie intake could cause the same effect as above, less spending on food could lead to more spending elsewhere, however, the effect on energy demand is uncertain. For example, if lower calories intake is combined with more spending on local, more expensive produce this could reduce the overall energy demand and offset the income effect. There has been comparatively less research on the rebound effect from reducing calories specifically, so it is uncertain the scale and direction of this rebound effect (Alfredsson, 2004; Tukker et al., 2011; Grabs, 2015).
Domestic buildings – thermal energy use
The domestic building sector focuses primarily on reducing the energy needed to heat UK homes. This is done through various assumptions/policies including improving the energy efficiency of homes (through retrofits or requirements for new build homes), deploying low carbon heating options, and changing heating habits through home working and smart heating systems. Rebounds can occur both directly (through higher internal temperatures rather than reduced energy use) or indirectly (by re-spending money saved on energy bills on other products). A summary of some relevant domestic rebound studies is given in Table 3, with a reported rebound range of 5–23%:
Reference | Modelled change | Type of rebound | Measure (e.g. GHG, energy etc.) | Rebound effect |
---|---|---|---|---|
Chitnis et al., 2013 | Household heating | Direct and indirect | CO2 emissions | 5–15% |
Chitnis et al., 2014 | Household heating | Direct and indirect | CO2 emissions | 6–23% |
Druckman et al., 2011 | Household heating | Direct and indirect | CO2 emissions | 7% |
Barker et al., 2007 | Household energy efficiency | Macroeconomic | CO2 emissions | 5–7% |
Household energy rebound studies have often focused on improved energy efficiency measures such as insulation or more efficient boiler installations. Other studies on low energy retrofits supports this by showing how household behaviour is an important determinant of energy demand reduction after retrofits (Ben and Steemers, 2014; Gupta and Gregg, 2015; Elsharkawy and Rutherford, 2018). Other studies also warn of ‘prebound effects’ in homes that do not achieve a high enough internal temperature pre-retrofit (often due to fuel poverty), therefore after the retrofit energy demand will remain and internal temperatures will increase (Teli et al., 2016). However, it is important to stress that although energy retrofits may under perform in terms of energy demand reduction, they can improve comfort households and are still important to improve quality of life.
Heat pumps are often more efficient than gas boilers and so there could be potential for similar rebound effects as above (Halvorsen et al., 2016). However, most of the current UK data on heat pump installation performance is from early adopter households that are not representative of the wider UK housing stock (Watson et al., 2021). Therefore, it is unclear how large the rebound may be for widespread heat pump adoption.
There is the potential that changes in heating habits (for example, heat as a service, smart metre initiatives) could offset rebounds by ensuring that internal temperatures do not change, and heat is only provided when needed. However, this could also create its own indirect rebounds if makes energy bills cheaper for households, but as with heat pumps, there is not enough research on this yet to draw solid conclusion (Watson et al., 2021).
The overall rebound effect of the domestic heating assumptions depends on several factors including behaviour, income, and current quality of housing so although the rebound from changes in domestic building heating is likely to be smaller than in other sectors (for example, transport or nutrition) there is still significant uncertainty.
Domestic buildings – lighting and appliances
A number of studies have examined the impact of replacing household lighting and appliances with more efficient technology, and as shown in Table 4, with a wide reported rebound range of 5-100%:
Reference | Modelled change | Type of rebound | Measure (e.g. GHG, energy etc.) | Rebound effect |
---|---|---|---|---|
Freire-González, 2011 | Household energy efficiency | Direct and indirect | Energy use | Short term – 56% Long term – 65% |
Davis et al., 2014 | Household appliances | Direct | Energy use | 72–100% |
Chitnis et al., 2013 | Efficient lighting | Direct and indirect | CO2 emissions | 5–15% |
Chitnis et al., 2014 | Efficient lighting | Direct and indirect | CO2 emissions | 5–17% |
The rebound effect depends largely on how households use their new appliances and how any money saved is re-spent and there are differences between the studies that could explain the range of rebound effects. These include: household income; how appliances are replaced and their cost; and what guidance is given to households (Mizobuchi, 2008; Chitnis et al., 2014; Elsharkawy and Rutherford, 2018).
The overall expected rebound effect depends on how appliances are replaced and how this demand is met. Inclusion of capital costs can reduce rebounds significantly because households spend initially on more efficient goods, which reduces how much money is saved. Therefore, if government schemes are deployed to encourage appliance replacement including subsidies, then there could be a substantial rebound effect as seen by Davis et al. (2014) where the replacement of appliances was subsidised for lower income households. Secondly new, more efficient appliances often contain more advanced, energy intense features (for example, an ice dispenser on a fridge) or if old, broken appliances are replaced and energy demand can actually rise (Davis et al., 2014). This can be mitigated by proper guidance to explain the most efficient usage of appliances (Elsharkawy and Rutherford, 2018) or by the distribution of smart meters but if these reduce household energy bills there is still a risk of indirect rebound effects and the overall effect of smart meters on rebound effects is more uncertain.
Lastly, no studies included the impact of energy efficiency measures on the wider economy and the replacement of inefficient appliances could generate economic growth through changes in production or re-spending and this could lead to other rebound effects.
Non-domestic buildings – thermal energy use
The non-domestic building sector includes two broad approaches to reducing energy demand, the first is deployment of energy efficiency measures to reduce heating energy demand and the second is a change to the growth expectations of floor space. The roll out of energy efficiency measures could result in similar rebound effects in non-domestic buildings as have been discussed for homes above, however, the rebound effect in non-domestic buildings has been comparatively less studied so the overall effect is uncertain.
There is an argument to be made that the direct rebound may be smaller in non-domestic buildings if heating timers/thermometers are set the same in office buildings that means that internal temperatures do not rise after energy efficiency retrofits. There could be more indirect or macroeconomic rebound effects but this depends on how businesses re-spend any savings and their carbon intensity.
Materials and products
For this sector the energy demand reduction scenarios aim to reduce embodied emissions from UK production using three methods:
- Energy efficiency – lower energy demand to produce each unit of output
- Resource efficiency – lower required output of materials and products, which in turn lowers associated energy demand
- Changes to the construction sectors demand for materials.
Large energy efficiency savings within UK industry is likely to result in macroeconomic wide rebounds effect by changing the production sector and encouraging economic growth. It is difficult to estimate exactly how large this rebound will be, however, a number of studies have shown large rebound effects (50% or higher and a number approaching 100% in the long run) (Berner et al., 2021; Bruns et al., 2021).
The rebound effect from resource efficiency improvements is less well studied than energy efficiency so there is more uncertainty about this. However, the same mechanisms will apply in terms of changes to production and the potential for economy-wide rebounds is similar to energy efficiency (Vivanco et al., 2018).
In terms of production, building materials are not constantly consumed (unlike electricity or water for example) therefore there is less incentive for consumption of these materials to increase after efficiency or cost savings (i.e. direct rebounds) (Bahn-Walkowiak et al., 2012). However, increased use of recycled materials could lead to bigger buildings if recycled materials are cheaper than non-recycled. This could also have an associated rebound by encouraging more construction. However, this could be offset by other assumptions within the scenarios that affect construction demand, for example the assumption that no new roads will be built or only small increases in demand for non-residential floor space.
Overall, there is potential for large macroeconomic rebounds within this sector, however, the combination of other sectors and assumption may reduce this.
Other cross-cutting trends and assumptions
There are other cross-cutting trends and assumptions that have not been covered specifically above but that could apply across multiple sectors. These could also have an impact on rebound effects.
- Digitalisation – This assumes that there will be improvements in efficiency across a number of sectors using digital technologies. However, the ICT sector is vulnerable to high rebound effects particularly in richer economies which may offset a significant portion of the efficiency savings (Lange et al., 2020).
- Sharing and circular economy – The effect of a circular economy on rebound effects is uncertain (Zink and Geyer, 2017). A circular economy can lead to a number of rebound effect either by substitution effect (for example, refurbished phones do not reduce demand for brand new phones but now creates demand for both) or the income effect (i.e. if refurbished/recycled goods are cheaper, then people can buy more of them). This also has the potential to encourage economic growth (Schulze, 2015).However, this can be offset by wider economic changes that would come along with a circular economy. For example, changes to consumer behaviour to buy more recycled goods instead of buying brand new through greater environmental awareness or education (reducing the substitution effect) or by targeting sectors with smaller price elasticity so that demand does not increase as much for a given price drop (reducing the income effect).
- Environmental awareness – This has been covered in other sectors, however, increased environmental awareness across the population could reduce rebound effects if it encourages people to spend their saved money, time etc. on lower energy demand or lower emitting options.
Conclusion
In general, the direct rebound from consumers energy services is around 10-30% in developed economies (Jenkins et al., 2017), however, studies that include more indirect and macroeconomic rebounds often find higher rebounds (Jenkins et al., 2017). Also, as shown above there is a large range between different sectors/services and some rebounds have been shown to be much higher including the potential for “backfire” (a rebound effect >100%) (Vivanco and van der Voet, 2014). Overall, the evidence is growing that economy-wide rebound is higher than once supposed, potentially above 50-60% (Brockway et al., 2021, Stern et al., 2021).
Certain sectors that are targeted in the low energy demand scenarios are vulnerable to significant rebound, particularly those that could have larger macroeconomic rebounds such as mobility, nutrition, and materials and products. Within these sectors there is potential for rebound effects between 50–100%. The other sectors are likely to experience much smaller rebounds but are still susceptible to rebound effects.
However, the rebound effects that are quantified within the literature are often based on isolated changes, based on the current economy and very few are based on actual policy interventions (Vivanco et al., 2018). The low energy demand scenarios present a wide range of policies and assumptions that combined could reduce the scale of the rebound. Secondly these scenarios expect large societal changes that could also mitigate rebounds, for example, living costs could decrease as a result of cheaper travel, and people may swap working for more leisure time which could reduce the pressure on economic growth and hence reduce rebounds.
Within the Transform demand scenario the UK could more than halve its energy demand by 2050 and reduce it by over 40% under the Shift demand scenario. If there is an average 20% rebound effect, then if UK aims for the Transform demand scenario they will still achieve an energy demand reduction the size of the Shift demand scenario. Also given that these scenarios aim to either maintain or improve standards of living, even a smaller reduction in energy demand is still a worthwhile cause. Although it is impossible to predict exactly how large the average rebound will be across the whole economy, an average rebound of 20% is feasible given the rebound effects within the literature and the potential mitigations.
Despite extensive research in some sectors, there is still a large degree of uncertainty of the rebound effect across all sectors, and this is highly dependent on the individual context of any changes. Therefore, there is a need for more detailed analysis of specific policies and assumption and ongoing monitoring if the recommendations of the low energy demand report are implemented. This is to ensure that rebounds effects are accounted and that further policies can be introduced where necessary.
References
Alfredsson, E.C. 2004. “Green” consumption—no solution for climate change. Energy, 29(4): 513–524. doi: 10.1016/j.energy.2003.10.013
Bahn-Walkowiak, B., Bleischwitz, R., Distelkamp, M. and Meyer, M. 2012. Taxing construction minerals: a contribution to a resource-efficient Europe. Mineral Economics, 25(1): 29–43. doi: 10.1007/s13563-012-0018-9
Barker, T., Ekins, P. and Foxon, T. 2007. The macro-economic rebound effect and the UK economy. Energy Policy, 35(10): 4935–4946. doi: 10.1016/j.enpol.2007.04.009
Ben, H. and Steemers, K. 2014. Energy retrofit and occupant behaviour in protected housing: A case study of the Brunswick Centre in London. Energy and Buildings, 80: 120–130. doi: 10.1016/j.enbuild.2014.05.019
Berner, A., Bruns, S.B., Moneta, A. and Stern, D.I. 2021. Do energy efficiency improvements reduce energy use? Empirical evidence on the economy-wide rebound effect in Europe and the United States. cege Discussion Papers. doi: 10.2139/SSRN.3854876
Bjelle, E.L., Steen-Olsen, K. and Wood, R. 2018. Climate change mitigation potential of Norwegian households and the rebound effect. Journal of Cleaner Production, 172: 208–217. doi: 10.1016/j.jclepro.2017.10.089
Brockway, P.E., Sorrell, S., Semieniuk, G., Heun, M.K. and Court, V. 2021. Energy efficiency and economy-wide rebound effects: A review of the evidence and its implications. Renewable and Sustainable Energy Reviews, 141: 110781. doi: 10.1016/j.rser.2021.110781
Bruns, S.B., Moneta, A. and Stern, D.I. 2021. Estimating the economy-wide rebound effect using empirically identified structural vector autoregressions. Energy Economics. 97: 105158. doi: 10.1016/j.rser.2021.110781
Budnitz, H., Tranos, E. and Chapman, L. 2020. Telecommuting and other trips: an English case study. Journal of Transport Geography, 85: 102713. doi: 10.1016/j.jtrangeo.2020.102713
Chitnis, M., Sorrell, S., Druckman, A., Firth, S.K. and Jackson, T. 2013. Turning lights into flights: Estimating direct and indirect rebound effects for UK households. Energy Policy, 55: 234–250. doi: 10.1016/j.enpol.2012.12.008
Chitnis, M., Sorrell, S., Druckman, A., Firth, S.K. and Jackson, T. 2014. Who rebounds most? Estimating direct and indirect rebound effects for different UK socioeconomic groups. Ecological Economics, 106: 12–32. doi: 10.1016/j.ecolecon.2014.07.003
Coulombel, N., Boutueil, V., Liu, L., Viguie, V. and Yin, B. 2019. Substantial rebound effects in urban ridesharing: Simulating travel decisions in Paris, France. Transportation Research Part D: Transport and Environment. 71: 110–126. doi: 10.1016/j.trd.2018.12.006
Davis, L.W., Fuchs, A. and Gertler, P. 2014. Cash for coolers: Evaluating a large-scale appliance replacement program in Mexico. American Economic Journal: Economic Policy, 6(4): 207–238. doi: 10.1257/pol.6.4.207
de Abreu e Silva, J. and Melo, P.C. 2018. Does home-based telework reduce household total travel? A path analysis using single and two worker British households. Journal of Transport Geography, 73: 148–162. doi: 10.1016/j.jtrangeo.2018.10.009
De Borger, B. and Mulalic, I. 2012. The determinants of fuel use in the trucking industry — volume, fleet characteristics and the rebound effect. Transport Policy, 24: 284–295. doi: 10.1016/j.tranpol.2012.08.011
Druckman, A., Chitnis, M., Sorrell, S. and Jackson, T. 2011. Missing carbon reductions? Exploring rebound and backfire effects in UK households. Energy policy, 39(6): 3572–3581. doi: 10.1016/j.enpol.2011.03.058
Elsharkawy, H. and Rutherford, P. 2018. Energy-efficient retrofit of social housing in the UK: Lessons learned from a Community Energy Saving Programme (CESP) in Nottingham. Energy and Buildings, 172: 295–306. doi: 10.1016/j.enbuild.2018.04.067
Freire-González, J. 2011. Methods to empirically estimate direct and indirect rebound effect of energy-saving technological changes in households. Ecological Modelling, 223(1): 32–40. doi: 10.1016/j.ecolmodel.2011.09.001
Gillingham, K.T. 2020. The rebound effect and the proposed rollback of U.S. fuel economy standards. Review of Environmental Economics and Policy, 14(1): 136–142. doi: 10.1093/reep/rez015
Grabs, J. 2015. The rebound effects of switching to vegetarianism. A microeconomic analysis of Swedish consumption behavior. Ecological Economics, 116: 270–279. doi: 10.1016/j.ecolecon.2015.04.030
Gupta, R. and Gregg, M. 2015. Do deep low carbon retrofits actually work? Energy Procedia, 78: 919–924. doi: 10.1016/j.egypro.2015.11.019
Halvorsen, B., Larsen, B.M., Wilhite, H. and Winther, T. 2016. Revisiting household energy rebound: Perspectives from a multidisciplinary study. Indoor and Built Environment, 25(7): 1114–1123. doi: 10.1177/1420326X16629725
Holden, E. and Linnerud, K. 2011. Troublesome leisure travel: the contradictions of three sustainable transport policies. Urban Studies, 48(14): 3087–3106. doi: 10.1177/0042098010396234
Jenkins, J., Nordhaus, T. and Shellenberger, M. 2011. Energy emergence: rebound and backfire as emergent phenomena. Oakland, USA: Breakthrough Institute.
Lange, S., Pohl, J. and Santarius, T. 2020. Digitalization and energy consumption. Does ICT reduce energy demand? Ecological Economics, 176: 106760. doi: 10.1016/j.ecolecon.2020.106760
Lenzen, M. and Dey, C.J. 2002. Economic, energy and greenhouse emissions impacts of some consumer choice, technology and government outlay options. Energy Economics, 24(4): 377–403. doi: 10.1016/S0140-9883(02)00007-5
Matos, F.J. and Silva, F.J. 2011. The rebound effect on road freight transport: Empirical evidence from Portugal. Energy Policy, 39(5): 2833–2841. doi: 10.1016/j.enpol.2011.02.056
Mizobuchi, K. 2008. An empirical study on the rebound effect considering capital costs. Energy Economics, 30(5): 2486–2516. doi: 10.1016/j.eneco.2008.01.001
Murray, C.K. 2013. What if consumers decided to all ‘go green’? Environmental rebound effects from consumption decisions. Energy policy, 54: 240–256. doi: 10.1016/j.enpol.2012.11.025
Raynaud, M., Osso, D., Bourges, B., Duplessis, B. and Adnot, J. 2016. Evidence of an indirect rebound effect with reversible heat pumps: having air conditioning but not using it? Energy Efficiency, 9(4): 847–860. doi: 10.1007/s12053-015-9419-2
Salemdeeb, R., Vivanco, D.F., Al-Tabbaa, A. and Zu Ermgassen, E.K. 2017. A holistic approach to the environmental evaluation of food waste prevention. Waste Management, 59: 442–450. doi: 10.1016/j.wasman.2016.09.042
Schulze, G. 2016. Growth within: A circular economy vision for a competitive Europe. Ellen MacArthur Foundation and the McKinsey Center for Business and Environment. pp.1–22.
Sorrell, S. 2007. The Rebound Effect: an assessment of the evidence for economy-wide energy savings from improved energy efficiency. London: UK Energy Research Centre. ISBN 1-903144-0-35
Sorrell, S. and Stapleton, L. 2018. Rebound effects in UK road freight transport. Transportation Research Part D: Transport and Environment, 63: 156–174. doi: 10.1016/j.trd.2018.05.006
Stapleton, L., Sorrell, S. and Schwanen, T. 2017. Peak car and increasing rebound: A closer look at car travel trends in Great Britain. Transportation Research Part D: Transport and Environment, 53: 217–233. doi: 10.1016/j.trd.2017.03.025
Stern, D.I. 2020. How large is the economy-wide rebound effect? Energy Policy, 147: 111870. doi: 10.1016/j.enpol.2020.111870
Teli, D., Dimitriou, T., James, P., Bahaj, A., Ellison, L. and Waggott, A. 2016. Fuel poverty-induced ‘prebound effect’ in achieving the anticipated carbon savings from social housing retrofit. Building Services Engineering Research and Technology, 37(2): 176–193. doi: 10.1177/0143624415621028
Tong, T.Y., Imamura, F., Monsivais, P., Brage, S., Griffin, S.J., Wareham, N.J. and Forouhi, N.G. 2018. Dietary cost associated with adherence to the Mediterranean diet, and its variation by socio-economic factors in the UK Fenland Study. British Journal of Nutrition, 119(6): 685–694. doi: 10.1017/S0007114517003993
Tukker, A., Goldbohm, R.A., De Koning, A., Verheijden, M., Kleijn, R., Wolf, O., Pérez-Domínguez, I. and Rueda-Cantuche, J.M. 2011. Environmental impacts of changes to healthier diets in Europe. Ecological Economics, 70(10): 1776–1788. doi: 10.1016/j.ecolecon.2011.05.001
Vivanco, D.F., Nechifor, V., Freire-González, J. and Calzadilla, A. 2021. Economy-wide rebound makes UK’s electric car subsidy fall short of expectations. Applied Energy, 297: 117138. doi: 10.1016/j.apenergy.2021.117138
Vivanco, D.F., Sala, S. and McDowall, W. 2018. Roadmap to rebound: how to address rebound effects from resource efficiency policy. Sustainability, 10(6): 2009. doi: 10.3390/su10062009
Vivanco, D.F. and van der Voet, E. 2014. The rebound effect through industrial ecology’s eyes: a review of LCA-based studies. The International Journal of Life Cycle Assessment, 19(12): 1933–1947. doi: 10.1007/s11367-014-0802-6
Watson, S.D., Lomas, K.J. and Buswell, R.A. 2021. How will heat pumps alter national half-hourly heat demands? Empirical modelling based on GB field trials. Energy and Buildings, 238: 110777. doi: 10.1016/j.enbuild.2021.110777
Wood, R., Moran, D., Stadler, K., Ivanova, D., Steen‐Olsen, K., Tisserant, A. and Hertwich, E.G. 2018. Prioritizing consumption‐based carbon policy based on the evaluation of mitigation potential using input‐output methods. Journal of Industrial Ecology, 22(3): 540–552. doi: 10.1111/jiec.12702
Zink, T. and Geyer, R. 2017. Circular economy rebound. Journal of Industrial Ecology, 21(3): 593–602. doi: 10.1111/jiec.12545
Banner photo credit: Thomas de Luze on Unsplash