2 Background
Reading time: 20 minutes
2.1 The need for global energy demand reduction
Globally, energy demand is increasing, driven by economic growth and the rising demand for energy services. For every 1% of additional Gross Domestic Product (GDP), energy demand increases by 0.68% (Brockway et al 2021). This is a long running trend dating back to 1971. Therefore, under current trends and given the ambition of every country to increase its GDP, energy demand is set to continue to increase.
This increase in global energy demand has occurred during a period of substantial energy efficiency improvements in all sectors. Efficiency of vehicles has improved year on year, improved insulation and better appliances continue to improve energy efficiency in buildings and industry continues to exploit energy efficiency options to reduce production costs. In a fossil fuel dominated energy system, improved energy efficiency has been the only significant downward pressure on global emissions (IPCC 2014, pdf), but its effect has been more than offset by rising population, incomes and energy demand increases from productivity improvements.
The importance of energy demand for the global energy system is clear. The higher the energy demand, the larger the size of the energy system and the slower the transition to carbon-free energy production. With growth in global energy demand, any additional renewable energy supply first has to meet new demand before it can displace fossil fuel production.
Energy demand also has major implications for assumptions about the reliance on CDR to achieve global climate goals. All but one of the scenarios presented in the IPCC’s 1.5ºC report rely on the use of large amounts (100 to 1,000 GtCO2) of CDR over the 21st century to achieve a 1.5ºC target with limited or no overshoot (Rogelj et al 2018, pdf). The exception relies on rapid and deep reductions in energy demand (Grubler et al 2018). Concerns about the feasibility and adverse impacts of the rapid roll out of CDR are well documented (Rogelj et al 2015; Anderson and Peters 2016; Smith et al 2016). In addition, it is not only the end point of net-zero emissions that matters but also the cumulative emissions on the pathway to it, as this determines the global temperature rise. Near term reductions in GHG emissions are therefore essential, and if CDR options do not materialise, earlier emissions are locked in, making climate goals unattainable.
Energy demand can be reduced in a number of ways: through energy efficiency improvements at the device level (e.g. vehicles, appliances), through delivering the same energy services for less energy (e.g. through modal shift from car to bus and increasing the use of recycled materials) and reducing the demand for energy services (e.g. by reducing the need to travel through local provision of services). Analysis has been undertaken at the global level on the potential contribution of lowering energy demand to achieve the global target of 1.5 degrees.
Grubler et al (2018) provides one of the most comprehensive assessments to date, constructing a scenario that would negate the use of CDR by transforming energy demand at the global level. The analysis shows that two world regions (global north and global south) will take different pathways to achieve the global target. However, the paper does not present the low energy demand scenario at the national level. Global scenarios provide a valuable framing, but not an operational plan to reducing energy demand to achieve global targets. National level action on energy demand reduction is essential as this is where most strategies and policies will need to be implemented. Key policies relating to travel demand, efficiency of appliances, retrofitting of buildings, resource efficiency and food waste reduction will all be implemented nationally, making it essential to understand how global potentials outlined in Grubler et al (2018) will be delivered.
We have categorised three ways to reduce energy demand.
- Avoid – Reducing the need for energy services: where this improves the quality of life. For example, this includes reducing unnecessary calorie intake, provision of local services to reduce the need to travel, food waste and lighting waste.
- Shift – Providing the same energy service differently: shifting calorie intake from meat to grain, shifting mobility from car to bus, shifting the purchasing of products to the services they provide.
- Improve – Reducing energy demand through energy efficiency: reducing the losses in converting delivered energy to useful energy. For example, adding more insulation to a wall or window, improving the efficiency of an electric transmission system, shifting heating from gas boiler to electric heat pump.
We recognise that there is considerable crossover between all three categories. For example, is a switch from a boiler to a heat pump an efficiency improvement or shift in technology? In reality, it is both. Our categorisation shown above is consistent with the approach described by Creutzig et al (2018) and commonly applied in the literature. The categorisation was used to ensure all options were considered, not to place different options within a fixed category.
2.2 The need for energy services
Whilst present levels of global energy demand are incompatible with limiting global temperature rises to less than 1.5ºC (without relying on speculative CDR technologies) (Grubler et al 2018; IPCC 2018), it is also important to recognise that many essential societal needs rely on the consumption of energy. It would be impossible to heat our homes, provide food, mobility and communication, recreation and leisure activities to name a few. Thus, the consumption of energy is essential to the satisfaction of important human needs. However, there is agreement in the literature that this relationship hits a saturation point, where increased energy consumption no longer leads to the further satisfaction of these needs (Steinberger and Roberts 2010; Burke 2020). This indicates that countries with low energy use and low human needs satisfaction (often in the global south) should be allowed to increase their energy use to fulfil these. But further, this relationship suggests that in high energy consuming nations that have surpassed this saturation point, like the UK, the potential exists to reduce energy consumption, whilst maintaining the satisfaction of human needs and quality of life (Vogel et al. 2021).
Fortunately, there are numerous additional benefits to reducing the UK’s energy demand that would improve or enhance quality of life while maintaining the services provided by energy. It is still possible to travel using alternative lower energy mobility (Brand et al 2019; Brand et al 2020). An active lifestyle is a healthy one while walking and cycling require negligible energy inputs in comparison to car travel (Brand et al 2021). Reducing travel demand where most people live improves air quality and creates safer neighbourhoods. A healthy diet can also be a low energy diet with additional benefits of reducing non-GHG emissions from livestock (Garvey et al 2021). A healthy diet can require less land area freeing up land for improving biodiversity while increasing recreational activities. Improving the fabric of a house to increase its efficiency results in warmer homes that use less energy. In addition, there are many new heating technologies that can reduce the operational costs for households. Businesses don’t want to consume energy but want the service it provides. Energy and resource productivity improvements in industry reduce costs and increase competitiveness.
While there could be negative implications of reducing energy demand, this clearly does not have to be the case. However, there are some intensive energy demand activities that would need to be addressed. Aviation is a good example of this. Fortunately, the energy demand associated with flights is concentrated in a relatively small high-income group meaning that most of the population would not be affected by any strategies to reduce travel demand for aviation. Research by Buchs and Mattioli (2021) suggest that between 2006 and 2017, 75% of flights were taken by 20% of the UK population and in a typical year around 50% will not fly at all. At the global level, 1% of the population causes 50% of the GHG emissions associated with aviation (Gössling and Humpe 2020). Car travel is also highly unequal, particularly in cities and towns where more than 30% of households do not own a car and car ownership is highly correlated with income (Brand et al 2013).
Figure 1 shows the distribution of energy demand across income groups in the UK, taken from Owen and Barrett (2020).
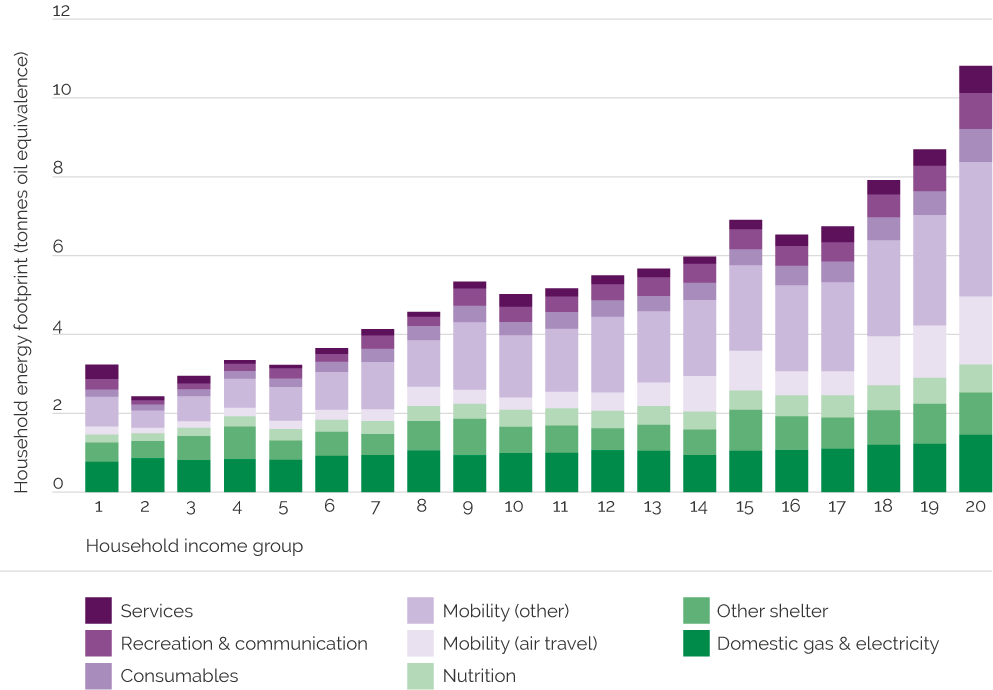
The analysis shows that the higher the level of income, the greater the energy demand. The difference between the lowest and highest energy using income group, is a factor of 4.5. This shows that it is possible to reduce energy demand in a way that does not increase levels of inequality. In fact, there is an opportunity to formulate a response to reducing energy demand while improving equality. One potential exception relates to household energy use for heating and power. The energy demand associated with home heat and power makes up a considerable proportion of low income energy demand. Any policies to reduce energy demand in this area will also need to reduce fuel poverty. Historically we have increased internal temperatures while reducing energy demand in our homes.
In summary, there are numerous co-benefits associated with reducing energy demand. Our low energy demand scenarios are designed to maximise these co-benefits, with one of our scenarios directly focusing on this issue.
2.3 UK energy demand
The UK Government currently has no comprehensive plan on how to reduce the UK’s energy demand. A comprehensive plan would include a clear projection of energy demand and a sense on how individual strategies and policies would reduce this demand over time. Without a reduction in energy demand, the burden is placed on an even more rapid roll out of renewables to meet high levels of energy demand as well as the reliance on technologies yet to be fully demonstrated e.g. carbon capture and storage.
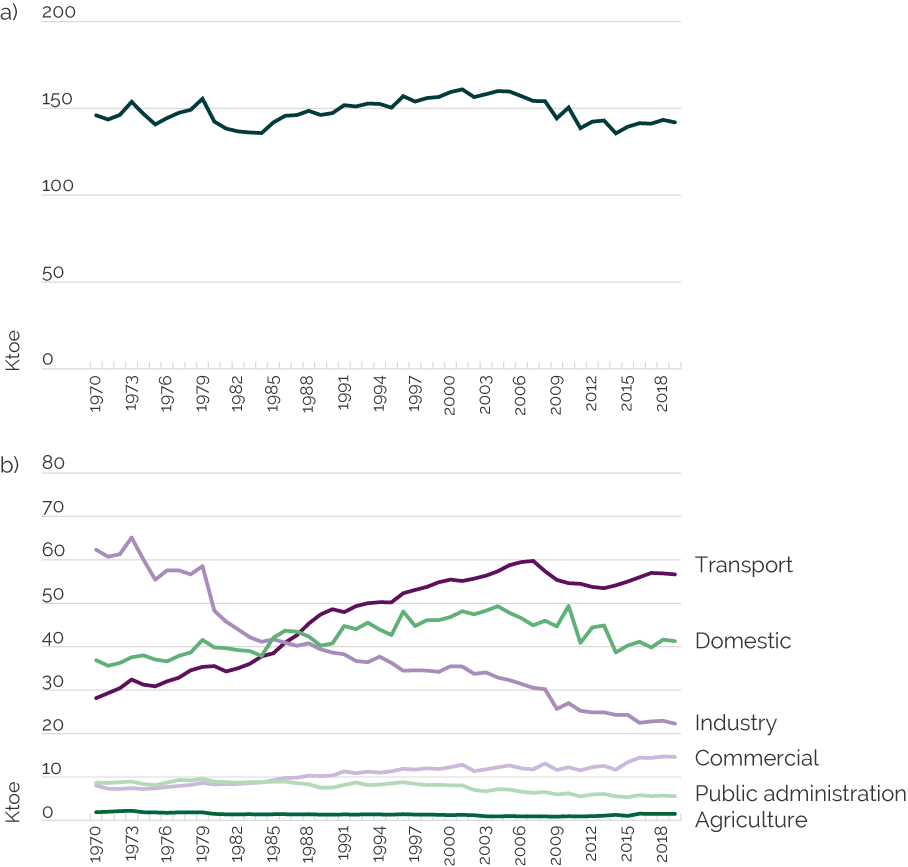
In the last 50 years, the UK’s energy demand has reduced by 2% (BEIS 2020b, pdf). In reality, there has been virtually no reduction in energy demand in the UK for 50 years. As can be seen in Figure 2, there have been increases and falls in the total energy demand over this time period. Energy demand was at its high point in 2001 (10% higher than 1971), since then there has been a decline with 2018 being 11% lower than in 2001. However, in recent years these reductions have ceased, with no notable reduction in UK energy demand for the past six years.
This has been driven by opposing effects in the demand for energy services and the efficiency with which they are delivered. In the last 30 years, energy efficiency improvements have made the largest contribution to reducing UK emissions, exceeding the combined effects of renewable energy and fuel switching from coal to gas (Lees and Eyre 2021).
The limited changes in total UK energy demand hide some significant changes in its sectoral composition. Energy demand for transport has doubled in the last 50 years while the population has only increased by 20%. Residential energy demand has increased by 11% over the same period while the number of households has increased by 47%, although this hides the fact that residential energy demand has reduced by 16% from its highest point in 2004. However, the reductions in residential energy demand have also slowed in recent years as energy efficiency programmes have weakened.
Energy demand for industry has reduced by 64% over the past 50 years. While some of this reduction can be attributed to energy efficiency improvements, the key driving factor is the shift in the UK towards a service-based economy (Hardt et al 2018). Data provided by the University of Leeds to the Department for Environment, Food & Rural Affairs (Defra) demonstrates that the UK’s demand for energy to satisfy UK consumption has increased, not reduced. However, the energy demand now occurs predominately outside the UK and is imported in the form of materials and products.
Future projections of UK energy demand undertaken by the Department for Business, Energy & Industrial Strategy (BEIS) suggest that energy demand will remain reasonably constant up to 2040, with their reference scenario showing a 2% decline over the next 20 years (BEIS 2020c). The BEIS analysis also suggests that known policies will have no significant effect on the overall level of UK energy demand. The analysis by BEIS refers to known policies, rather than future policy aims or targets.
2.4 Relevant analysis of energy demand scenarios at international and global levels
This section identifies studies that have explored the potential mitigation contribution of reducing energy demand. The following literature review highlights that whilst there is a good understanding of mechanisms through which energy demand can be reduced and the potential range of impact this could have upon future global cumulative GHG emissions, there remains a limited picture of how energy demand reduction could be implemented at the national level, or the contribution it could make towards meeting national climate targets.
2.4.1 Energy efficiency-led scenarios
The low energy demand (LED) scenario developed by Grubler et al. (2018) is a frequently cited study that explores the potential contribution of global reductions in energy demand to GHG mitigation. Operating at the global level, it projects that energy demand could be 40% lower than today by 2050, based on a bottom-up analyses of changes in activity levels, energy intensity and final demand of end-use energy services. Its inclusion in the IPCC’s (2018) Special Report on 1.5ºC offered a contrasting perspective to other 1.5ºC aligned scenarios, as the only scenario that did not increase energy demand. By reducing global energy demand, the reliance on speculative CDR measures to achieve ambitious carbon budgets was diminished (Rogelj et al 2018, pdf). Furthermore, Grubler et al. (2018) evidence that reducing the size of the global energy system makes the task of transitioning to a low carbon supply much easier to achieve, as low carbon energy technologies can assume a larger share of the total energy mix.
The IEA’s (2020) Energy Technology Perspectives 2020 report, included energy demand reducing measures within its Sustainable Development Scenario, in the form of exploiting energy efficiency improvements present in technologies. Broadly speaking, the scenario covers two groups of efficiency improvements, both of which are included in our UK focused LED scenario, adopting technologies that carry with them reductions in energy intensity, and material efficiency that aims to reduce energy service demand by reducing the material inputs needed to produce goods and services. Similarly to Grubler et al (2018), it argued that energy efficiency measures that reduce overall energy use are crucial to decarbonisation of energy systems, as it reduces the ‘resource constraint’ problem with the roll out of low carbon technology (IEA 2020).
Brugger et al (2021) assess the potential for overarching societal trends to have an impact on energy efficiency and final energy demand at the EU level. They identify 12 societal trends that may have a future impact on the success of energy efficiency policies and future energy demand, both positive and negative. These societal trends relate to digitalisation, new social and economic models, industrial transformation, and changes in quality of life. In a best-case scenario, trends such as increased consumer awareness, urbanisation and smaller space living reduce energy demand by 67% compared with an EU baseline projection of final energy demand. Conversely, their worst-case scenario sees new societal trends contributing to a 40% increase in energy consumption through increased efficiency rebounds. They suggest this wide range of impacts displays that energy efficiency gains do not by themselves lead to energy demand reduction, highlighting the need for strong policy frameworks that ensure energy efficiency gains have the desired effect (Brugger et al 2021).
2.4.2 Changes to social practices, lifestyles, and behaviour
The literature also includes scenarios that explore the extent that energy demand could be reduced through changes to how society consumes energy services. There are various terms used to describe this mechanism for demand reduction, such as ‘lifestyle change’ (Eyre et al 2009; van Sluisveld et al 2016; van Vuuren et al 2018; Institute for Global Environmental Strategies & Aalto University 2019, pdf), ‘behaviour change’ (IEA 2020; Niamir et al 2020) or social change (Grubler et al 2018; Kuhnhenn et al 2020; Ivanova et al 2020).
Van Vuuren et al (2018) explore global scenarios consistent with limiting warming to 1.5ºC that minimise the reliance on CDR strategies. Their scenario includes significant energy demand reduction through ‘lifestyle change’ including reduced meat consumption, modal shift in mobility service provision, changes in heating preferences and the avoidance of consumption of household appliances. Whilst on their own, these changes are found to be unable to match the pace of mitigation required, as part of a broader mitigation pathway including energy efficiency measures and shifts to low carbon energy production, demand reduction measures significantly reduce the need for CDR in limiting warming to 1.5ºC.
Eyre et al (2009) develop a scenario of lifestyle change in the UK built upon increasing concerns about energy use and its environmental implications. They suggest that lifestyle change impacts service demands mostly in residential and transportation energy services and have the potential to reduce national energy use and carbon emissions by 35% and 30% respectively. They conclude that such a shift would reduce the cost of transitioning the energy supply to low carbon alternatives by £70 billion, concurring with others in the literature (Grubler et al 2018; IEA 2020) that energy demand reduction helps to facilitate supply-side transitions.
The Social Transformation Scenario developed by Kuhnhenn et al (2020) is the most transformative scenario in terms of reducing energy demand. It does so by reducing the demand for high carbon goods and services, focusing on high carbon freight and passenger transport, heating, energy consuming appliances, and food. Energy demand in countries in the global north is significantly reduced through a strong decline in overall consumption levels, creating room for increased necessary consumption in the global south, whilst reducing global energy demand and staying within a global carbon budget consistent with 1.5ºC of warming. They argue that the global north has a responsibility to dramatically reduce its consumption of high carbon goods and services, and abandon the pursuit of economic growth, to facilitate the dual aim of redistributing wealth within nations and internationally, whilst achieving mitigation rates consistent with limiting warming to 1.5ºC.
Keyßer and Lenzen (2021) assess global 1.5ºC scenarios that include a general reduction in economic output in the global north, due to strong climate mitigation. They compare these ‘degrowth’ mitigation scenarios to IPCC ‘archetype scenarios’ that assume ever expanding growth in economic output and energy demand. Whilst the political feasibility of degrowth is questioned, they suggest a reduction in final demand levels reduces the reliance on decoupling GDP from energy use at unprecedented rates, the development of unpredictable CDR technologies, and the unmatched speed of technological change necessary to decarbonise the current the energy supply.
Ivanova et al (2020) evaluate the extent that various sustainable energy service consumption options led to genuine GHG mitigation, across food, housing, transport, and other consumption. They find that their top 10 most effective consumption options have the potential to mitigate 9.2 tCO2eq/cap, compared to the high carbon alternative consumption option. They suggest this indicates an untapped area of mitigation that goes beyond discussions of the efficiency of production, to consider the nature of and scale of consumption in relation to planetary boundaries and satisfying human needs.
Modelling social change using integrated assessment models is a key challenge discussed widely in the literature, with various approaches being taken (van Sluisveld et al., 2016; Niamir et al., 2020; Sharmina et al 2021). Van Sluisveld et al (2016) insert ‘lifestyle change’ measures into an integrated assessment model, by changing key model parameters based on estimates established in the literature. Whilst this simple method does not directly capture agent decision making in the model, it facilitates a quick estimation of the scale of reductions given lifestyle changes could facilitate. Niamir et al. (2020) address this flaw, integrating an agent-based modelling framework into a computable general equilibrium model. This facilitates the scaling up of agent-based models of heterogeneous individuals to a larger level, capturing regional, social-demographic, and structural differences in individual’s decision making when adopting low carbon decision making. Whilst these modelling approaches and methods used throughout the literature represent significant improvements in the methodologies to model social change-led energy demand reduction and GHG mitigation, the need for further model development is a key suggestion for further research throughout the literature.
2.4.3 Sectoral energy demand reduction
Some of the literature explores energy demand reduction scenarios in individual sectors with significantly high embodied energy such as construction (Mata et al 2020), agriculture (Poore and Nemecek 2018; Garvey et al., 2021), transport (Khalili et al 2019) and other hard to mitigate sectors such as aviation, shipping, freight, and industry (Sharmina et al., 2021). Mata et al. (2020) investigate energy demand reduction in the building sector, through an exploration of sector roadmaps for net-zero around the world. They find that most roadmaps are focused on efficiency measures and technology-based upgrades to the housing stock, with limited focus on ‘lifestyle’ or ‘behavioural’ changes (Mata et al., 2020). Brand et al. (2019) explore four contrasting futures that compare transport-related ‘lifestyle’ changes and socio-cultural factors against a transition pathway focusing on transport electrification and the phasing out of conventionally fuelled vehicles. They find that lifestyle change alone can have a comparable and earlier effect on transport carbon and air quality emissions than a transition to electric vehicles with no lifestyle change.
Sharmina et al. (2021) identify key demand reduction options in four critical ‘hard to reach’ sectors: aviation, shipping, road freight and industry. They highlight that currently, integrated assessment models are unable to capture many demand side mitigation opportunities within their price elasticity demand mechanisms, such as modal shift for aviation, slow stemming for shipping, localised production reducing demand for freight transport, and circular economy measures in industry. Khalili et al. (2019) investigate scenarios of future global transport energy demand, mapping the shift away from fossil fuelled transport. They suggest that the efficiency improvements accompanying low carbon fuel switching and electrification could offset the projected increase in global demand for passenger and freight transport, and this increased demand can be managed by a stable final energy demand in 2050, compared to 2015.
The literature that centres reductions in energy demand to support transitions to low carbon energy supply offers a crucially important alternative to those dominant scenarios relying solely on a supply-side transition and CDR. Given the pace and urgency needed to successfully mitigate the worst impacts of the climate crisis and the uncertain capacity of CDR measures to mop up remaining emissions, reducing final energy demand in countries where it is high is likely to be crucial to achieve the goals of the Paris Agreement.
However, to date, energy demand reduction scenarios have operated at a multi-national or global level, whilst mitigation targets and climate policies are devised at a national level, through Nationally Determined Contributions (NDCs) and net-zero commitments. This presents a gap in the energy demand reduction scenario literature that has direct policy relevance. This demand for tailored national-level evidence highlighting the capacity of energy demand reductions as a mitigation solution, is present in the Climate Change Committee’s Sixth Carbon Budget (2020b), including energy and material efficiency measures, as well as changes to social practices including diet switching and modal shifting in transport. To further support national demand side climate mitigation policy, research is needed to develop cohesive frameworks for nations to put into operation energy demand reduction measures given unique national contexts. This research aims to fill this gap by developing a tailor-made low energy demand scenario for the UK.